-
Lithium-ion batteries – electrolytes – solid & semi-solid
-
ALL-SOLID-STATE CELL
Applicant:
TOYOTA MOTOR /
US 2025125490 A1
All-solid-state cells with sulfide electrolyte and lithium metal negative electrodes were constructed.
Cells with 0.25 vol% Sn in the first solid electrolyte
layer exhibit a charging capacity of 1.65 mAh/cm2 when
charged between 3.0 V and 4.2 V at 3 mA/cm2. This optimal cell included an
Mg layer (thickness: 1000 nm) deposited on the Ni foil current collector and a Sn layer
(thickness: 100 nm) between the Mg layer and the first solid electrolyte layer.
The outstanding performance is attributed to the optimal metal phase proportion that allows
for effective trapping of lithium dendrites while maintaining good ionic conductivity.
The Li2S ∙ P2S5 ∙ LiI solid electrolyte
serves as both the first and second solid electrolyte layers, with only the first
layer containing the dispersed Sn phase.
When charged, an alloy of Sn and Li forms in the first solid electrolyte layer, functioning
as a protective layer that prevents decomposition of the sulfide solid electrolyte,
resulting in superior cycling characteristics without short circuits.
The Figure below shows a schematic cross-sectional view of an all-solid-state cell with the
following layers: 1: negative electrode current collector (Ni foil), 2: metal layer (Mg metal), 3A: first solid electrolyte
layer (containing Sn metal phase), 3B: second solid electrolyte layer (Li2S ∙ P2S5 ∙ LiI),
4: positive electrode active
material layer, 5: positive electrode current collector, 10: all-solid-state cell, DT: thickness
direction.
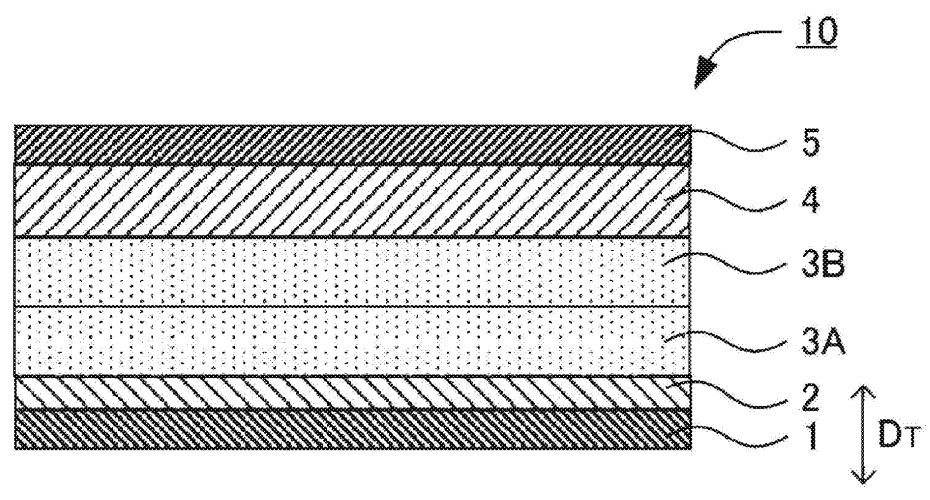
This work illustrates how the presence of Sn and Mg at the lithium metal / sulfide electrolyte interface reduces the risk of lithium
dendrite formation while charging all-solid-state lithium metal cells with sulfide electrolytes.
-
The premium version includes another two patent discussions, plus an Excel list with 50-100 commercially relevant recent patent families.
-
Lithium-ion batteries – negative electrode (excluding Li metal electrodes)
-
A silicon-carbon negative electrode material was prepared by a two-step process. In
the first step, porous carbon was heated (500°C, 2 h, under Ar, rotary kiln)
to remove adsorbed oxygen. After vacuum evacuation to
1×10-5 Pa and argon purging, monosilane and argon (volume ratio 1:4) were
introduced to form silicon-carbon particles with 200 Pa micropositive pressure, with the
kiln rotating at 40 Hz.
In the second step, the temperature was raised to 600°C and a gas mixture of
acetylene, methane, and argon (volume ratio 2:1:7) was introduced at 0.2 kPa
pressure (40 Hz rotation).
The resulting material exhibits a high-valence silicon content of 15.2% in the
region extending 10 nm inward from the surface, 7.4% at 10-20 nm depth, and
2.9% at 20-30 nm depth, as determined by X-ray photoelectron spectroscopy (XPS)
depth profiling using argon ion etching. XPS analysis also confirms the distribution of low-valence
silicon (binding energy 98-102 eV) and high-valence silicon (binding energy
102-106 eV, see Figure).
In contrast, the XPS spectrum of the comparative example processed at 200°C exhibits
a much higher proportion of high-valence silicon, with the high-valence silicon
peak (102-106 eV) being more prominent relative to the low-valence silicon peak
(98-102 eV).
The resulting material exhibits a discharge capacity of 1,984 mAh/g and a
first cycle efficiency of 93.8% in half-cell tests. In contrast, the comparative example processed at 200°C exhibits
a
lower discharge capacity of 1,766 mAh/g, a reduced first cycle efficiency of
83.5% in half-cells.
强度: intensity
低价硅: low-valence silicon
高价硅: high-valence silicon
基线: baseline
电子结合能: electron binding energy (eV)
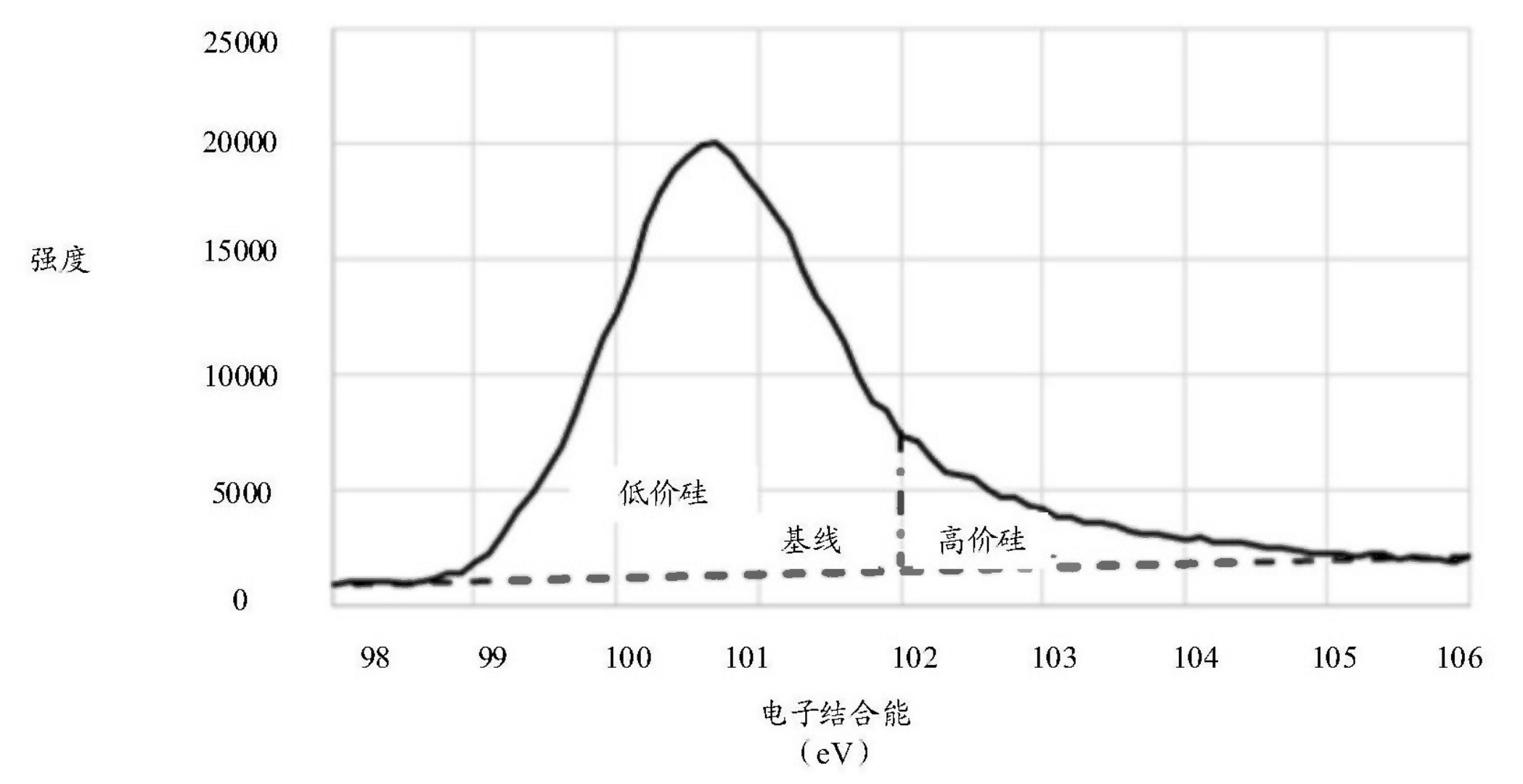
The difference in silicon valence states between samples can potentially be explained by
oxidation mechanisms. Low-valence silicon (0 to 2+ oxidation states) represents
silicon species like elemental Si (0) and partially oxidized silicon, while
high-valence silicon (3+ to 4+ oxidation states) presumably consists of silicon
oxide species like SiO2. When processed at insufficient temperatures (200°C),
oxygen from residual gases in the reaction vessel or from the carbon framework
itself might react with deposited silicon, forming silicon oxides. At the optimal
temperature (600°C), the carbon coating deposition rate is presumably higher and more
uniform, creating a protective layer that effectively prevents silicon oxidation.
XPS measurements in Si-carbon composite materials might also explain electrochemical differences
in other Si-carbon materials that appear similar in terms of other characterization methods
(such as Si content & crystallinity, particle size distribution, BET specific surface area).
-
The premium version includes another two patent discussions, plus an Excel list with 50-100 commercially relevant recent patent families.
-
Lithium-ion batteries – positive electrode
-
ANIONIC REDOX HIGH ENERGY CATHODES
Applicant:
WILDCAT DISCOVERY TECHNOLOGIES / TIANJIN B & M SCIENCE TECHNOLOGY /
WO 2025065466 A1
Li1.15Mn0.6Ni0.225O2 was doped with tungsten and coated with
lithium borate (LBO). The core material was prepared by ball milling lithium,
manganese, and nickel precursors (selected from Mn2O3, Mn3O4,
Ni(OH)2, Li2CO3) in a planetary mill using zirconia media (5 mm
size, 6 h). The milled precursors were annealed (870°C, 10 h, air)
to form the lithium-rich manganese nickel oxide
material.
For tungsten doping, the precursors were modified to achieve a composition of
Li1.15Mn0.596Ni0.224W0.005O2 (W-containing precursor not identified).
The lithium borate
coating was applied by ball-milling H3BO3 (1 mol%) with the active
material in ethanol/water (7:3 by mass) using Teflon
milling media. The solid content was maintained at 25 mass%. The resulting mixture
was dried (90°C, 2 h) and annealed (500°C, 5 h, air) to form the LBO
coating.
For comparison, an uncoated (pristine) Li1.15Mn0.6Ni0.225O2
material, a material with only LBO coating (1 mol%), and a material with only
Nb2O5 coating (2 mol%) were prepared using similar procedures. The
Nb2O5 coating was applied using Nb(OC2H5)5 as the
precursor.
The coated and uncoated materials were used to prepare cathodes (90 mass% active
material, 5 mass% Super C65 carbon black, 5 mass% PVDF) with a loading of
11.1 mg/cm2. Full cells were assembled with graphite anodes and tested with
a standard electrolyte (EC/EMC/LiPF6, 2% FEC, 6% DEC, 2 mass%
tris(trimethylsilyl) phosphite).
As shown in Figure below, the
W-doped LBO-coated material exhibits the best performance, maintaining a
cycling capacity above 190 mAh/g after 200 cycles. The LBO-coated and
Nb2O5-coated materials exhibit similar performance with capacities around
185 mAh/g after 200 cycles, while the uncoated pristine material rapidly degrades
to below 100 mAh/g after approximately 150 cycles.
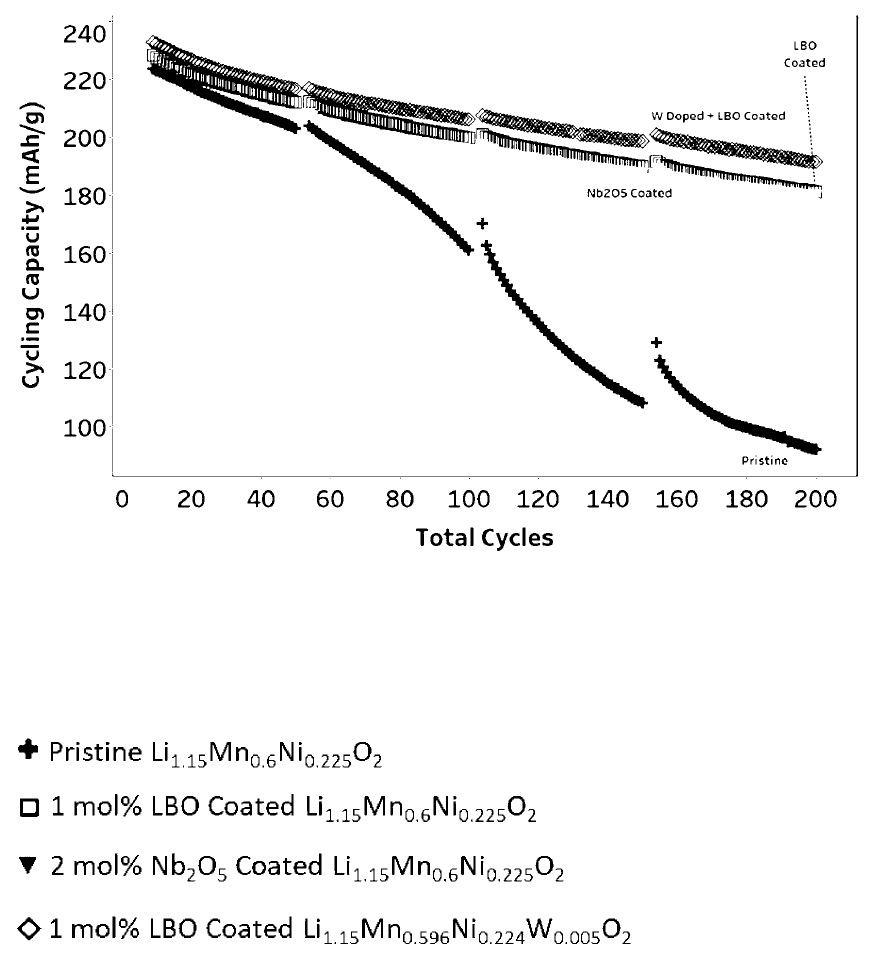
This work illustrates how the combination of tungsten doping and lithium borate coating improves the cycling stability of
LRLO (Li-rich layered oxide) active materials.
-
The premium version includes another two patent discussions, plus an Excel list with 50-100 commercially relevant recent patent families.
-
Fuel cells (PEMFC / SOFC / PAFC / AEMFC) – electrochemically active materials
-
A PEMFC electrode was prepared with a cathode catalyst layer comprising
three sequentially arranged layers (first, second, and third catalyst layers), with
the first catalyst layer adjacent to the proton exchange membrane. Each catalyst layer
independently contains catalyst and ionomer, with the first catalyst layer containing
hydrophilic nanoparticles and the third catalyst layer containing hydrophobic
nanoparticles.
The hydrophilic nanoparticles in the first catalyst layer includes TiO2
nanoparticles with a specific surface area of 100-400 m2/g. The mass ratio
of hydrophilic nanoparticles to catalyst is 0.05-0.8:1.
The second catalyst layer contains only catalyst and ionomer without any additional
nanoparticles, serving as a transition layer between the hydrophilic first layer and
the hydrophobic third layer. The Pt loading in the second layer is 0.05-0.15
mg/cm2, similar to the other catalyst layers.
The hydrophobic nanoparticles in the third catalyst layer include PTFE nanoparticles
with a specific surface area of 100-400 m2/g. The mass ratio of hydrophobic
nanoparticles to catalyst is 0.02-0.40:1.
The catalyst used in all three layers is TEC10E50E (Tanaka Precious Metals) consisting
of 50.5% Pt supported on 49.5% carbon black. This commercial Pt/C catalyst was used
in its as-received state without further modification. In the experimental examples,
the catalyst was dispersed in water and mixed with isopropanol and Nafion D2020 ionomer
solution (20% solid content) to form the catalyst ink for each layer.
This design results in a hydrophilic-to-hydrophobic gradient from
the membrane side outward, improving both proton conduction near the membrane and
formation of capillary force gradients in catalyst layer pores, facilitating oxygen
transport and liquid water removal, thereby enhancing fuel cell power density.
The electrode layers were applied sequentially by ultrasonic spray-coating onto a Gore
proton exchange membrane (12 μm thickness), with each layer dried (70°C, 5 min)
before applying the next layer. The anode catalyst layer was applied to the opposite
side of the membrane with a Pt loading of 0.05 mg/cm2.
As shown in the Figure, the polarization curve comparison between Example 1 (with the
three-layer gradient design) and Comparative Example 1 (conventional catalyst layer)
demonstrates significant performance improvements across the entire current density
range. The performance enhancement is particularly pronounced at high current densities,
indicating improved mass transport capabilities of the gradient cathode structure.
对比例1: comparative example 1
实施例1: example 1
电压(V): voltage (V)
电流密度(A/cm2): current density (A/cm2)
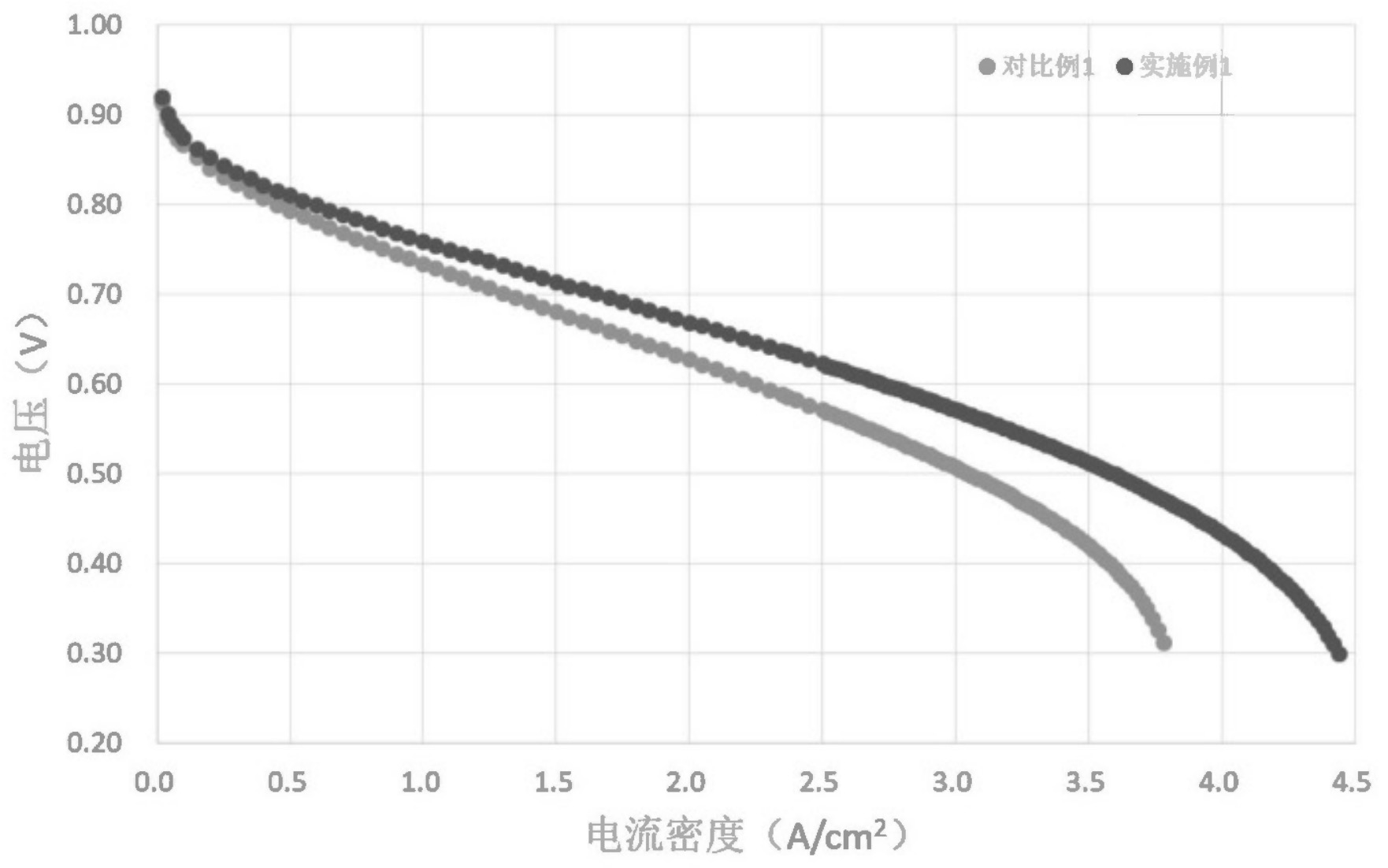
This work illustrates how a gradient structure in the cathode catalyst layer of a PEMFC can improve performance, through
the selective incorporation of TiO2 and PTFE nanoparticles.
-
The premium version includes another two patent discussions, plus an Excel list with 50-100 commercially relevant recent patent families.
-
Triweekly patent lists for other categories (Excel files are included for premium users)
-
- Lithium metal batteries (excluding Li-S, Li-Air): XLSX
-
- Lithium-air batteries: XLSX
-
- Lithium-ion batteries – electrolytes – liquid: XLSX
-
- Lithium-ion batteries – separators: XLSX
-
- Lithium-sulfur batteries: XLSX
-
- Na-ion batteries: XLSX
-
Prior patent updates
-
2025-04-29
-
2025-04-08
-
2025-03-18
-
2025-02-25
-
2025-02-04
|